New antidiabetic drugs' role in the management of testosterone deficiency and of the cardiovascular disease in hypogonadal diabetic men
Abstract
The known hallmarks of type 2 diabetes mellitus (T2DM), such as hyperglycemia, insulin resistance, visceral adiposity, inflammation, endothelial dysfunction, and oxidative stress, are known to influence the hypothalamus-pituitary-gonadal axis, leading to functional hypogonadism. Both the consequent testosterone (T) deficiency and diabetes are recognized factors influencing cardiovascular (CV) risk. In this context, T replacement therapy showed an improvement in glycemic control and metabolic, anthropometric, and body composition parameters in hypogonadal diabetic individuals. Observational and randomized studies on T replacement therapy suggested the beneficial effect of this treatment on CV risk, although inconclusive results should still be evaluated, particularly when subgroups of patients have to be considered. In this setting, the novel antidiabetic drugs have demonstrated beneficial effects on T levels, due to their positive effects on the hypothalamic–pituitary–gonadal axis, in addition to a proven CV protective action. Thus, the combined metabolic and CV effects of T replacement therapy and novel antidiabetic drugs are of great interest. In this review, we aimed to summarize the present state of the art concerning the association between T deficit and CV risk in diabetic people by analyzing the relationship between endogenous T and CV system in diabetic men. In particular, the impact of novel antidiabetic drugs on male hypogonadism, and the combined cardio-metabolic effects of T supplementation and novel antidiabetic drugs were discussed.
Keywords
INTRODUCTION
Diabetes mellitus (DM) is recognized as a condition at high cardiovascular (CV) risk, regardless of previous CV events. In particular, type 2 DM (T2DM) is usually accompanied by additional CV risk factors, such as obesity, hypertension, dyslipidemia, nonalcoholic fatty liver disease (NAFLD), and inflammation. Hyperglycemia, insulin resistance (IR), visceral adiposity, inflammation, endothelial dysfunction, and oxidative stress together represent the well-known hallmarks of T2DM. These conditions often alter the hypothalamus-pituitary-gonadal (HPG) axis function culminating in the development of functional hypogonadism consisting in low testosterone (T) circulating level and, in the long-term, in erectile dysfunction, reduced semen quality, and infertility as well[1-3]. Furthermore, functional hypogonadism could also lead to poorer glycemic control, lower insulin sensitivity, as well as increased fat mass in abdominal region due to the reduction of androgen-induced lipolytic effect[4]. Thus, T2DM is generally associated with hypogonadism, which, in turn, contributes to CV risk in diabetic males.
Hypogonadism in adult men is a clinical condition characterized by a decreased functional activity of the testes, resulting in decreased production and/or action of androgens and/or impaired sperm production[5]. The hypogonadism diagnosis requires serum T levels lower than the normal range (confirmed twice), together with the presence of specific clinical manifestations[5,6]. However, no validated serum T cut-off level has been established, but different thresholds have been proposed so far. Thus, either total T serum levels < 12 nmol/L (350 ng/dL), or T < 11 nmol/L (320 ng/dL) in middle-aged and elderly men[7,8] could be considered to define hypogonadism[5,8-10]. In general, T serum levels lower than 8 nmol/L (230.5 ng/dL) are widely accepted to define severe hypogonadism[5,9,10]. In the gray zone between 8 and 10-12 nmol/L, the presence of hypogonadism-related symptoms should be checked to confirm the diagnosis. In particular, sexual dysfunction (i.e., reduced libido, reduced spontaneous or stimulated penile erection, and erectile dysfunction), hot flashes, reduced semen volume, and decreased hair in androgen-dependent areas are the main signs and symptoms detectable [Figure 1].
Figure 1. Evaluation and management of Testosterone Deficiency. TD: testosterone deficiency; TT: total testosterone; LH: luteinizing hormone; SHBG: sex hormone binding globulin; PRL: prolactin; TRT: testosterone replacement therapy.
Hypogonadism is strongly associated with metabolic disorders such as obesity, hypertension, diabetes, and dyslipidemia. Clinically, T deficiency is correlated with increased fat mass, reduced insulin sensitivity, impaired glucose tolerance, and elevated triglyceride and total cholesterol[11]. In particular, the association between DM[1], prediabetes[12] and male hypogonadism has been largely claimed and proven. In addition, many studies showed that T deficiency per se is an independent risk factor of CV and all-cause mortality[13], likely due to the direct effects of T on myocardial and vascular structure and function. Moreover, considering hypogonadism treatment and CV safety in the general population, and in particular in DM, the evidence from literature is still inconclusive. In this context, the potential effect of androgen therapy on the CV risk of males with DM remains far from being completely elucidated. With this in mind, the objectives of the review are to investigate: (1) evidence and controversies of T treatment and the CV risk in diabetes; (2) impact on male hypogonadism of novel antidiabetic drugs with proven CV protective effect; and (3) combined cardio-metabolic effects of T supplementation and novel antidiabetic drugs.
DIABETES AND MALE HYPOGONADISM: A BIDIRECTIONAL RELATIONSHIP
Epidemiology
Several cross-sectional studies consistently demonstrated that subnormal T serum levels are common in men with diabetes, regardless of the type[14-20]. Overall, hypogonadism prevalence in males with T2DM ranges from 26% to 48%, considering total T (TT) serum levels[21-26], and from 19% to 57%, evaluating free T (FT)[21,22,24,25,27,28]. Thus, it could be expected that approximately more than one-third of T2DM men suffer from hypogonadism. Although hypogonadism is a common pathophysiological condition that occurs with increasing age, T deficiency has been observed in T2DM men also before 35 years[28], and already at the time of T2DM diagnosis[25], suggesting that this association is evident at a young age when diabetes is present. Accordingly, the prediabetes condition results are also associated with hypogonadism, with an overall calculated average prevalence ranging from 24% to 35%[12]. On the contrary, T deficiency prevalence seems to have a lower prevalence in type 1 DM (T1DM). The studies conducted in these subjects revealed a prevalence of hypogonadism ranging from 0 to 9.5% considering TT[21,22,24,28-30], and 3%-22% when FT levels were evaluated, respectively[21,22,24,28]. However, limited information is available on latent autoimmune diabetes in adults (LADA), in which hypogonadism prevalence is scantly reported, accounting for 8.2% of cases in one report[31].
As a confirmation of this association, whether hypogonadism is a common finding in diabetic men, a potential protective role of T on T2DM development has been suggested so far. Indeed, higher T serum levels in men seem to be protective against T2DM[32] and prediabetes[33] risk.
Overall, lower T serum levels in diabetics are generally associated with reduced gonadotropin (Gn) production, representing hypogonadotropic hypogonadism[27,34]. Only several diabetic subgroups populations show either T deficiency with inappropriately normal follicle-stimulating hormone (FSH) and luteinizing hormone (LH)[14], or high gonadotropins serum levels in subgroups of individuals with diabetic neuropathy[35], the latter representing hypergonadotropic hypogonadism.
Why are diabetes mellitus and hypogonadism associated?
The IR represents one of the main pathogenetic mechanisms of diabetes, at least considering T2DM. At the same time, several studies reported an association between IR and low T serum levels in men[36-38]. In line with this mutual influence, the relationship between DM and hypogonadism appears to be bidirectional[39].
From a molecular point of view, T and insulin can influence each other. Indeed, T leads to a non-genomic activation of several signaling factors which contribute to insulin receptor functioning, such as protein kinase B (Akt), extracellular signal-regulated kinase (ERK), and mechanistic target of rapamycin (mTOR), increases the expression of insulin receptor beta subunit (IR-β), insulin receptor substrate 1 (IRS-1), glucose-transporter-type 4 (GLUT4), and glycolysis enzymes[40]. Moreover, T increases the GLUT4 expression in cultured skeletal muscle cells, hepatocytes, and adipocytes[41], as well as membrane translocation, promoting glucose uptake in adipose and skeletal muscle tissue[42]. According to these in vitro studies, low T serum levels were found to induce IR in vivo[43,44]. Alongside these systemic T effects, the potential effect of this steroidal hormone on pancreatic beta cells is still not completely unraveled. Indeed, while some studies reported an increased androgen receptor-dependent hyperglycemic decomposition[45], other authors described a protective role[46].
By contrast, insulin has a role in restoring T and Gn serum levels[39]. In particular, insulin may increase the synthesis and the release of Gn-releasing hormone (GnRH) from the hypothalamus, and it may directly stimulate T secretion[47].
Low T serum levels increase adipogenesis and visceral obesity, which is associated with IR, chronic inflammation, and low sex hormone binding globulin (SHBG) levels[48]. This finding is in line with the positive effect of dihydrotestosterone on lipid tissue: it can inhibit lipid accumulation in human subcutaneous, mesenteric, and omental preadipocytes[49]. However, it is known that visceral obesity is more common in men than in premenopausal women[50]. In addition, it was found that androgen binding sites are two times higher in intra-abdominal preadipocytes than in subcutaneous preadipocytes[51], and that there is a direct positive transcriptional effect of androgens on the expression of the antilipolytic α2A-adrenoreceptor subtype in rodent mature adipocytes[52]. Nowadays, the role of androgens on body composition is still not completely understood.
SHBG is a protein secreted by the liver that, thanks to its steroid hormones binding property, has important regulatory actions on the levels and activity of steroid hormones, such as testosterone, dihydrotestosterone, and estradiol[53]. However, SHBG is also a protective molecule against metabolic syndrome (MetS), thanks to its suppressive action on adipocyte inflammation and lipid accumulation. Indeed, low levels of SHBG can be used as a marker of MetS, hepatic steatosis development, and IR, and also as a predictor of T2DM. As a matter of fact, chronic low-grade inflammation diseases, including obesity, IR, and T2DM, can decrease SHBG levels[54].
Increased fat mass seems to further reduce serum T. Adipose tissue converts circulating T into estradiol (E2) since it expresses the aromatase enzyme. An increase in serum E2 further contributes to a decrease in serum T since E2 exerts the main inhibitory effect (negative feedback) on Gn secretion both at hypothalamic and pituitary levels[55]. Conversely, IR might occur first (especially in obese diabetic patients), and then can lead to increased adipose tissue, triggering hypogonadism[56]. In particular, insulin and insulin-like growth factor 1 signaling are adipogenic, and as we described before, adipose tissue mass and its distribution can affect the circulating concentrations of T[57]. However, this is just one of the potential mechanisms linking adipose tissue excess and hypogonadism. Indeed, even more demonstrations suggested that the correlation between obesity and hypogonadism is bidirectional, also sustained by leptin dysregulation and systemic inflammation[58,59]. Thus, in this complex scenario, other factors could lead to hypogonadism in diabetic subjects, such as the resistance to GnRH-stimulating effects of leptin in patients with obesity, the suppressive action of leptin on the stimulatory action of Gn on the Leydig cells, and finally, the increase of inflammation that leads to the suppression of hypothalamic GnRH secretion[60-62].
Finally, increased glucocorticoid activity in adipose tissue also appears to induce androgen inactivation due to increased activity of 11β-hydroxysteroid dehydrogenase type 1, leading to decreased androgen activity in adipose tissue[63] [Figure 2].
MALE HYPOGONADISM AND CARDIOVASCULAR RISK
It is well known that men have a higher CV risk compared to premenopausal women, suggesting a protective role of estrogens and, likely, a negative role of T on CV health. Recently, this hypothesis has been questioned because some evidence documented a relationship between hypogonadism, metabolic status, and CV risk. Indeed, low T serum levels were detected as a predictive marker for ischemic arterial diseases, such as coronary heart disease and stroke[64], and for CV-related mortality[65-67]. A recent meta-analysis confirmed this relationship, quantifying the association between low T levels and CV morbidity and mortality[68]. Indeed, CV risk and mortality were inversely related to mean age, and directly related to the prevalence of diabetes and the proportion of active smokers[68]. This association is particularly true in diabetic men, in which low T serum levels significantly predicted all-cause mortality during long-term follow-up[69]. However, the mechanism behind this correlation has not been completely understood. Studies in animal models suggest that low T could be involved in the regulation of inflammation in several tissues[70-73], worsening CV health. In humans, T has a vasodilatory effect through the downregulation of L-type voltage-gated calcium channels and the upregulation of calcium-activated potassium channels, increasing the cardiac contractility and cardiomyocyte relaxation, reducing the atheroma development, and reducing the lipid deposition in the artery wall[39]. Moreover, T decreases the production of inflammatory cytokines, such as tumor necrosis factor-alfa, interleukin-1b and interleukin-6, and increases the anti-atherogenic interleukin-10 levels[47]. Finally, T seems to have a weak anticoagulant activity, stimulating tissue factor pathway inhibitor and tissue plasminogen activator expression, and inhibiting plasminogen activator inhibitor type 1 secretion by the endothelium[47]. Alongside the direct T action on the endothelium, T could improve CV health, influencing the metabolic profile and reducing the risk of obesity, IR, metabolic syndrome, DM, and lipid profile impairment, which are widely reported in association with male hypogonadism[74]. As a confirmation, subjects treated with androgen deprivation therapy showed an increased risk of DM and coronary artery diseases[75], myocardial infarction[75,76], CV mortality and sudden cardiac death[77,78], and heart failure[79].
TESTOSTERONE TREATMENT AND CARDIOVASCULAR HEALTH IN DIABETES
It is largely demonstrated that hypogonadal men should be treated with exogenous T, also to prevent/reduce CV risk[80]. T replacement therapy improves sexual function[81,82], increases skeletal muscle mass[82,83], strength[84], and bone mineral density[82,83], and ameliorates lipid profile[85,86] and IR[43]. These effects are extremely beneficial in young men with hypogonadism, while in older men, the benefits and long-term risks must be properly evaluated[87].
Considering CV health, little evidence described a detrimental effect of T replacement therapy, increasing CV risk[88,89] and adverse outcomes[90], in particular in older men or in younger subjects with pre-existing heart diseases[89]. According to these controversies about the effect of T replacement therapy on CV risk, the US Food and Drug Administration (FDA) added restrictions through a warning statement on this substitutive treatment. However, these reports must be carefully considered, and recent analyses confirmed that, when prescribed according to the recommended dosage, T replacement therapy in hypogonadal patients improves angina symptoms in subjects with ischemic heart disease and exercise ability in patients with heart failure[91]. Obviously, the potential benefits of T treatment in reducing CV risk should be examined in longer-term trials with specific designs[68]. Indeed, T replacement therapy has been evaluated in specific conditions, such as men with heart failure, in whom T administration increases the cardiac output acutely[92] and improves functional capacity and symptoms[93], apparently via reduction of left ventricular afterload and/or change in cardiac morphology. Similarly, a beneficial effect has also been suggested in men with moderate/severe heart failure, in whom the supplementation of long-acting T resulted in improving functional capacity and baroreflex sensitivity for control of heart rate, together with the improvement of muscle strength and glucose metabolism[94]. With this in mind, exogenous T administration seems to be overall beneficial on the CV risk, particularly when TT basal levels were below 12.1 nmol/L. In these men, T-based therapy resulted in high patient satisfaction and reduced CV-related mortality[95].
The lack of a clear demonstration of the long-term safety of T replacement therapy on CV health led to confusion about when this treatment should be considered in hypogonadal men with T2DM. Indeed, many of the studies available in the literature evaluating the safety profile of T on CV health were not specifically designed for the diabetic population. Thus, the same considerations of the general population should be applied to diabetic men. Specifically considering diabetes, Muraleedharan et al. documented a significant survival improvement in 581 men with T2DM treated with T for six years[69]. Moreover, a randomized clinical trial has been designed with the purpose of answering the question of whether T therapy is associated with an increase in CV events. The testosterone replacement therapy for assessment of long-term vascular events and efficacy response in hypogonadal men (TRAVERSE) is a randomized, double-blind, placebo-controlled, parallel-group, non-inferiority, multicenter study that will determine the CV safety and long-term efficacy of exogenous T in middle-aged and older men with hypogonadism with or at increased risk of CV disease[96].
The association between T replacement therapy and CV risk in men with T2DM must consider other influencing factors. Indeed, the T action on CV risk could be mediated by the indirect effects on glycemic control, comorbidities, and additional diabetes-related CV risk factors[97]. As a confirmation, potential glucose metabolism improvement after androgen replacement therapy is suggested in diabetic men, allowing the reduction of glycated hemoglobin (HbA1c), fasting plasma glucose and homeostasis model assessment of insulin resistance[85,98-102]. In addition, T supplementation seems able to improve the metabolic parameters in individuals with hypogonadism and metabolic syndrome[103] or prediabetes[12]. Indeed, androgen therapy restores the expression and phosphorylation of the adenosine 5’-monophosphate-activated protein kinase-α, a mediator of exercise-induced glucose uptake in skeletal muscle, contributing to insulin sensitivity and glucose homeostasis improvement[104]. Moreover, T replacement therapy in diabetic men improves lipid profile, reducing total cholesterol, triglyceride, low-density lipoprotein (LDL) cholesterol, and lipoprotein A, and increasing high-density lipoprotein cholesterol[101,102]. T supplementation shows a beneficial effect on blood pressure control, with a specific reduction of diastolic blood pressure in obese men[105], and an overall decrease in blood pressure in T2DM[101,106]. Finally, T replacement therapy in diabetic men improves anthropometric[101,107] and body composition parameters[43,101,106], inflammatory markers[43,108], and endothelial function[109,110]. Thus, all these demonstrations, although still sparse and not conclusive, suggest that T replacement therapy in T2DM men with hypogonadism contributes to CV risk improvement both directly and indirectly through the modulation of other influencing factors.
IMPACT OF NOVEL ANTIDIABETIC DRUGS WITH PROVEN CARDIOVASCULAR PROTECTIVE EFFECT ON MALE HYPOGONADISM
Considering the bidirectional link between T deficiency and DM-related complications, such as CV risk and mortality[4,111-113], hypoglycemic agents have been expected to exert a positive impact on HPG-related dysfunction in diabetic men.
Metformin represents one of the first hypoglycemic oral agents developed with favorable metabolic benefits. Beyond the well-known better glycemic control, accumulating evidence ascribed multiple functions to this drug, among these anti-obesity, renal/cardioprotective and anticancer roles[114]. For these reasons, metformin is still used as the first step drug in the management of glucose control in T2DM in combination with novel hypoglycemic agents[115,116]. Even in the presence of proven metabolic control and cardiovascular protection, experimental results described an anti-androgenic role of metformin as well as a negative impact on testicular and reproductive health[117,118].
Human studies reported the harmful effect of metformin on T production. Men with newly diagnosed T2DM, after rapid glycemic normalization by short-term intensive insulin treatment, showed the recovery of T levels in a eugonadal state whose concentrations were lowered when further exposed to metformin for 1 month[119]. Similarly, prolonged duration of metformin-based therapy reduced T levels and counteracted the T elevation accompanied with the improvement of blood glucose[120]. Low T levels have also been observed in patients under metformin regardless of age, duration of the disease, and HbA1c[121]. Notably, a recent study demonstrated that when fathers took one or more prescriptions for metformin during the development of fertilizing sperm, the likelihood of their male offspring having genital birth defects was increased[122].
These findings collectively indicated that the use of metformin may be another reason for the high prevalence of low T and reproductive abnormalities in males with T2DM. However, the mechanisms underlying the dangerous effects of metformin on human testicular health are still poorly clarified.
Conversely, new glucose-lowering agents have demonstrated a positive impact on body weight, waist circumference, hyperglycemia, atherosclerosis, and potentially on the HPG axis as well.
Glucagon-like peptide-1 receptor (GLP-1R) agonists (GLP-1Ra) and sodium-glucose cotransporter protein-2 inhibitors (SGLT2i) are currently used in T2DM management. GLP-1Ras are known to exert pleiotropic effects, including body weight control, glycemic control, and CV protection as well[123-125]. In accordance, SGLT2i inhibit renal glucose reabsorption, ameliorating blood glucose levels with additional effects on body weight and blood pressure, leading to improved renal and CV outcomes in subjects with T2DM, especially heart failure and kidney failure[126-131]. Thus, these drugs are efficient in protecting diabetic male patients from several players of disturbances of the HPG axis (i.e., advanced glycation end-products, reactive oxygen species, etc.)[132]. Considering the association between HPG failure and cardiometabolic outcomes, as well as a potential vasoprotective role of T replacement therapy in hypogonadal men[133], particularly with erectile dysfunction[132,134], a possible CV and metabolic benefit of T-based therapy is reasonable. However, conflicting results have been obtained regarding the effects of T replacement therapy on the occurrence of major adverse CV events, as will be discussed further[90,135-137]. Based on these considerations, herein we discuss current evidence about the effects of GLP-1Ra and SGLT2i on HPG function in hypogonadal diabetic males. Moreover, a potential additive effect on cardiometabolic outcomes from the combined administration of T replacement therapy and hypoglycemic agents will be further discussed.
Glucagon-like peptide-1 receptor agonists
Recent findings highlighted that GLP-1R is located in several cells related to the HPG axis as well, suggesting a potential GLP-1Ra effect on the HPG axis. Indeed, GLP-1R genetically abrogated in male mice resulted in poor development of gonads and seminal vesicles without modifications in the number and distribution of gonadotrophic cells within the anterior pituitary and in reproductive behavior[138]. However, GLP-1R is widely expressed in various central areas as hypothalamic nuclei orchestrating the release of GnRH and LH, and more recently was also identified in testicular cells[139]. Moreover, in vitro exposure of a neuronal cell line (GT1-7) to GLP-1 caused an increase in intracellular cAMP, together with the enhanced release of LH-releasing hormone[140]. This effect has also been confirmed in male rats where intracerebroventricular injection of GLP-1 induced a prompt increase in circulating levels of LH[140]. Further, in vitro analysis observed that increasing doses of GLP-1 stimulate hypothalamic GnRH neurons by enhancing the mRNA levels of kisspeptin-1 (KISS-1), an HPG axis gatekeeper, together with increased GnRH mRNA expression, effects inhibited in the presence of a selective GLP-1R antagonist[141].
The role of GLP-1 on gonadal function was elucidated by in vivo model of ischemia/reperfusion-induced testicular dysfunction. In this model, mice undergoing testicular torsion showed enhanced levels of oxidation (i.e., malondialdehyde, 3-nitrotyrosine), inflammatory [i.e., hypoxia-inducible factor-1β, tumor necrosis factor-α (TNF-α), etc.], and proapoptotic markers (i.e., caspase3, etc.) in association with downregulation of KISS-1 and its receptor (KISS-1R) in testis[142]. Notably, treatment with semaglutide before reperfusion alleviated dysfunction, inflammation, and oxidative stress of testis, probably due to the restoration of KISS-1 expression, which in turn improved testicular energy production and utilization[142]. Semaglutide injection also restored steroidogenesis pathway-related genes (i.e., steroidogenic acute regulatory protein, cytochrome P450 family 11 subfamily a member 1, etc.) and increased the expression of proliferating cell nuclear antigen in testicular cells, representing the key protein involved in DNA damage repair[142].
Effects on semen quality of GLP-1Ras have also been observed in obese male mice. This model of diet-induced obesity is typically characterized by impaired sperm quality through increased DNA damage, increased testicular inflammation (due to a rise in TNF-α, and monocyte chemoattractant protein-1), and abnormal sperm physiology[143]. All these conditions seem to be reversible after exenatide treatment[143].
Recent results support the functional role of the GLP-1/GLP-1R system in testicular somatic cells as well[144]. Indeed, GLP-1R has recently been identified in human Sertoli cells whose exposure to low doses of GLP-1 increased energy utilization, while at the highest concentration, it reduced mitochondrial membrane potential and oxidative damage[144]. Moreover, Rago et al. demonstrated that GLP-1Ra could directly ameliorate the seminal plasma quality since the expression of GLP-1R has been found in human spermatozoa[145]. Indeed, human sperm cells exposed to increasing concentrations of exenatide showed a significant increase in progressive motility and cholesterol efflux occurring through the activation of the cAMP/protein kinase a (PKA) pathway[145]. In concert, these findings suggest that GLP-1Ra-based therapy may bring additional advantages, improving the inflammatory status of testis and sperm quality and function not only directly by favoring GLP-1R activation in spermatozoa, but also indirectly through the activation of the GLP-1R in Sertoli cells.
Liraglutide, semaglutide and dulaglutide, the long-acting formulations, have demonstrated significant cardiovascular protective effects. In particular, liraglutide mitigated the risk of CV death[146], while semaglutide and dulaglutide conferred a risk reduction on non-fatal stroke[147,148]. Similar results were obtained by the Exenatide Study of Cardiovascular Event Lowering (EXSCEL) trial, where exenatide ameliorated major adverse CV events even though to a less extent as compared with long-acting drugs[149-151]. These effects seem to be particularly mediated by an anti-atherogenic effect of GLP-1Ra as confirmed by animal models of hyperglycemia where liraglutide-based therapy reduced lipid deposition and plaque volume on the aortic surface according to glucose levels amelioration[152].
Based on the anti-atherogenic effects of GLP-1Ra, a possible action of these drugs on erectile dysfunction is reasonable. In this regard, Yuan et al recently demonstrated that liraglutide could improve erectile function in diabetes-induced erectile dysfunction by regulating smooth muscle relaxation, oxidative stress and autophagy, independently of the glucose-lowering effect, in a rat model of type 1 diabetes[153]. In addition, the supplementation of liraglutide to metformin therapy ameliorated endothelial functions of corpus cavernosum of male obese subjects with T2DM, resulting in the recovery of erectile performance[154]. Similar results were also obtained by the Researching Cardiovascular Events with a Weekly Incretin in Diabetes (REWIND) trial, where the long-term treatment with dulaglutide was also found to reduce the incidence of moderate or severe erectile dysfunction in middle-aged men with T2DM[155]. These beneficial effects on erectile function are probably mediated also by the recovery of normal T production. Indeed, incretin mimetics appear to bring T levels into the eugonadal range, as shown when exenatide-based therapy was combined with glimepiride or metformin treatment in hypogonadal middle-aged men with T2DM and obesity[156]. Similar results were obtained in a prospective randomized open-label study where the treatment of obese men with liraglutide induced a significant increase in TT serum levels (+ 2.6 ± 3.5 nmol/L) together with the improvement of LH and FSH secretion[157]. Thereafter, a recent retrospective study conducted in obese diabetic men with hypogonadism reported that the weight loss obtained with either liraglutide or dulaglutide, rather than the glycemic control, is the main driver of the improvement of T levels (≥ 300 ng/dL)[158]. Nevertheless, the beneficial testicular effects of these emerging therapies appear to vary according to basal levels of androgens, as emerged in a recent prospective cohort study where the exenatide-based therapy did not significantly change FT levels in diabetic men without hypogonadism[159], thus supporting a relevant role of incretin mimetics on male HPG axis, particularly in hypogonadism. Previously, experimental observations in healthy men documented no effect of GLP-1 on the pattern of LH secretion, but with intravenous infusion of physiological, low doses of GLP-1[160].
In summary, GLP-1Ra-based therapy may potentially act on each player of the HPG axis, fostering LH secretion by hypothalamic-pituitary neurons, T production by testis, ameliorating the semen quality and improving erectile function [Figure 3 and Table 1]. Further elucidations are needed to clarify whether these effects are mediated by indirect actions of GLP-1Ra on glycemic control, body weight, inflammation, hormonal variation, or by direct interactions of these drugs with GLP-1R in different areas of the HPG axis.
Figure 3. Potential direct and indirect effects of GLP-1Ra and SGLT2i on HPG axis function, CV system function and insulin resistance in diabetic hypogonadal men. FSH: follicle-stimulating hormone; LH: luteinizing hormone; GnRH, Gonadotropin-releasing hormone; GLP-1Ra: glucagon-like peptide-1 receptor agonist; KISS-1: kisspeptin-1; SGLT2i: sodium-glucose cotransporter protein-2 inhibitor; HPG: hypothalamus-pituitary-gonadal.
Sodium-glucose cotransporter protein-2 inhibitors
Recent in vivo findings ascribed endothelial and anti-atherogenic functions to SGLT2i in terms of enhanced cardiac muscle remodeling, decreased vascular stiffness, and preventing the development of heart failure as observed in mice models of diabetes[161,162]. Similarly, studies conducted in diabetic patients reported that dapagliflozin-based therapy significantly improved systemic endothelial function and reduced both renal resistive index and aortic stiffness[163].
Despite the proven beneficial effects of SGLT2i in the setting of different diabetes-related cardiometabolic disorders, the contribution of these novel compounds to HPG axis function is still poorly explored. Data from a diabetic rat model with erectile dysfunction showed favorable effects of empagliflozin on erectile function, as compared to placebo, when the hypoglycemic treatment was followed by acute sildenafil administration[164]. This improved erectile response under SGLT2i was due to an increased cavernosal nitrergic relaxation, suggesting a positive effect of empagliflozin on the nerve injury[164]. Moreover, testicular benefits with SGLT2i treatment were demonstrated in leptin receptor-deficient diabetic mice, where the administration of dapagliflozin improved seminiferous tubule destruction, increased sperm concentrations and motility and protected testicular cells from apoptosis, increasing B-cell leukemia/lymphoma 2 protein and X-linked inhibitor of apoptosis protein, and oxidative stress, through the increase of superoxide dismutase and glutathione peroxidase activity[165]. Furthermore, dapagliflozin enhanced circulating levels of GLP-1 and the expression of GLP-1R within testicular tissue in a phosphatidylinositol-3 kinase (PI3K)/Akt-dependent manner [165]. Nevertheless, these effects on gonadal structure and sperm quality were partially lost after administration of GLP-1R antagonist exendin (9-39), thus suggesting that dapagliflozin may protect against diabetes-induced spermatogenic dysfunction via GLP-1R/PI3K/Akt-dependent pathway[165].
To date, only one human retrospective study demonstrated benefits on the HPG axis associated with SGLT2i-based therapy, highlighting that treatment with dapagliflozin increased T secretion in obese patients with uncontrolled T2DM and hypogonadism[158]. The enhanced T production observed was explained because of the amount of weight loss and the reduction in testis inflammation[158].
Even though the effects of SGLT2i on T synthesis are still poorly appreciable, in vivo experiments suggest a potential protective action of these drugs against cavernosal nerve alterations and sperm dysfunction [Table 1].
Experimental findings supporting the actions of the novel antidiabetic drugs on hypothalamic-pituitary-gonadal axis and erectile function
GLP1-Ra | SGLT2i | ||||||||
Experimental studies | Human trials | Experimental studies | Human trials | ||||||
baseline | post-therapy | baseline | post-therapy | ||||||
Hypothalamus-pituitary function | In vitro ↑ GnRH[140,141] ↑ KISS-1[141] | LH (IU/L) [2.7 ± 1.2] FSH (mIU/L) [4 ± 1.7][157] | LH (IU/L) [3.4 ± 1.1] FSH (mIU/L) [4.9 ± 2.1][157] | None | None | ||||
In vivo ↑ LH[140] ↑ KISS-1[142] | |||||||||
Gonadal function | In vivo Beneficial effect on steroidogenesis pathway-related genes[142] Beneficial effect on DNA damage repair system[140,142,143] ↓Inflammation[143] ↓ oxidative damage[144] Effect on seminal quality (↑ in progressive motility)[145] | TT (nmol/L) [7.6 ± 1.5][157] TT (ng/dL) [262 ± 11][158] | TT (nmol/L) [10.2 ± 4.2][157] TT (ng/dL) [> 300][158] | In vivo Effect on seminal quality (sperm concentrations and motility)[165] ↓ oxidative damage[165] Protect spermatogenic dysfunction via GLP-1R/PI3K/Akt-dependent fashion[165] | TT (ng/dL) [265 ± 11][158] | TT (ng/dL) [> 300][158] | |||
Erectile function | Beneficial effects on smooth muscle relaxation, oxidative stress, and autophagy[153] | Improved ADAM and AMS after therapy[156] | Beneficial effect on erectile dysfunction by ↑ cavernosal nitrergic relaxation[164] | None |
COMBINED CARDIO-METABOLIC EFFECTS OF TESTOSTERONE REPLACEMENT THERAPY AND NOVEL ANTIDIABETIC DRUGS
Several prospective cohort studies evaluated the association between endogenous T levels and the risk of CV disease. An inverse correlation was noted in the presence of severe hypoandrogenemia when the risk of CV death (RR 1.25, 95%CI: 0.97-1.60) and all-cause death (RR 1.35, 95%CI: 1.13-1.62) was higher in the setting of low T synthesis[166]. However, increasing endogenous T levels were significantly correlated with the decreased risk of CV death, with men in the highest quartile having an odds ratio of 0.53 (95%CI: 0.32-0.86) compared with men in the lowest quartile[67]. Therefore, low serum levels of endogenous T represent a risk factor for CV events, CV mortality, and all-cause mortality[167]. A similar association was also observed between gonadal functions and metabolic disturbances. Indeed, men with T deficiency have increased IR and low glucose tolerance regardless of age[43,44], as well as an increased risk of developing diabesity[168,169]. Indeed, Ding et al. showed that men with T concentrations above 15.5 nmol/L (447 ng/dL) had a 42% reduced risk of T2DM compared to men with T levels below 15.5 nmol/L[32]. The presence of hyperglycemia and reduced insulin sensitivity during T2DM could contribute to exacerbating HPG failure in hypogonadal men, thus sustaining an endless detrimental loop[132].
Considering the tight association between the disruption of the HPG axis and T2DM-related complications, the therapeutic efficacy of T replacement therapy on cardiometabolic outcomes is reasonable. As a matter of fact, Kapoor et al. reported that the administration of 100 mg/week of T for 3 months reduces HbA1c by 0.37%, fasting blood glucose by 1.58 mmol/L, total cholesterol by 0.4% and visceral fat in hypogonadal men with T2DM[170]. In line with this evidence, after 24 weeks of T replacement therapy, diabetic men with functional hypogonadism ameliorated insulin sensitiveness in terms of upregulation of key insulin signaling genes (IR-β, IRS1, AKT-2 and glucose transporter protein type-4) in the subcutaneous adipose tissue together with the improvement of lipid and inflammatory profile[43]. In contrast, a randomized clinical trial did not observe any improvement in glycemic control and IR as well as in visceral fat area in diabetic hypogonadal men when T replacement therapy was administered for a short period[85,107,171]. However, by extending the duration of T treatment to 2 years, both parameters achieved normal values[109,172].
The heterogeneity of study populations and differences in the duration of therapy could interfere with the real CV effectiveness of T replacement therapy. Since the effects of T on CV system are still debated, the cardiometabolic role of combined T and hypoglycemic therapies is still far from being investigated. Nevertheless, in a retrospective observational study conducted in men suffering from T2DM and overt hypogonadism, the supplementation of liraglutide to T replacement therapy allowed a consistent body weight reduction to be achieved and glycemic targets to be reached, together with a recovery in androgen levels[154]. However, in this study, no CV events have been reported in the medical history of patients enrolled[154]. Hence, further randomized, placebo-controlled studies with large cohorts of patients are needed to elucidate the effectiveness of co-administration of T and antidiabetic drugs on cardiometabolic outcomes in diabetic men with overt hypogonadism.
CONCLUSION
Clinical data on the effects of T treatment on CV outcomes produced contradictory and/or inconclusive results so far. However, in this context, it is essential to keep in mind the possibility that the risk is dose-dependent or higher in certain groups, such as the elderly. The trial TRAVERSE will address the uncertainty regarding CV safety of T replacement therapy among middle-aged or older men with or at high risk for CV disease. Taking into consideration diabetic people, impairment of glucose homeostasis and low T levels are strongly associated. In particular, in T2DM men, lowered serum T predicts DM-related comorbidities, high CV risk, and increased mortality. Furthermore, exogenous T showed beneficial effects on glycemic control and overweight/obesity, IR, dyslipidemia, hypertension, inflammation and endothelial dysfunction, all these recognized comorbidities and additional CV risk factors in T2DM. In this context, the novel GLP-1Ra and SGLT2i antidiabetic drugs have shown preliminary evidence of effects on T levels, in addition to a proven CV protective action [Figure 3]. The combined metabolic and CV effects of T replacement therapy and novel antidiabetic drugs are of great interest, and therefore requires further appropriately designed studies.
DECLARATIONS
AcknowledgmentsThe authors thank Professor Manuela Simoni for her precious scientific support.
Authors’ contributionsConception or design: Greco C
Drafting the work or revising: Greco C, Genchi V, Zanni E, Colzani M, Lauriola C
Final approval of the manuscript: Greco C, Genchi V, Zanni E, Colzani M, Lauriola C, Cignarelli A, Santi D
Availability of data and materialsNot applicable.
Financial support and sponsorshipNone.
Conflicts of InterestAll authors declared that there are no conflicts of interest.
Ethical approval and consent to participateNot applicable.
Consent for publicationNot applicable.
Copyright© The Author 2023.
REFERENCES
1. Dhindsa S, Ghanim H, Batra M, Dandona P. Hypogonadotropic hypogonadism in men with diabesity. Diabetes Care 2018;41:1516-25.
2. Maresch CC, Stute DC, Alves MG, et al. Diabetes-induced hyperglycemia impairs male reproductive function: a systematic review. Hum Reprod Update 2018;24:86-105.
3. Genchi VA, Rossi E, Lauriola C, et al. Adipose tissue dysfunction and obesity-related male hypogonadism. Int J Mol Sci 2022;23:8194.
4. Zheng R, Cao L, Cao W, et al. Risk factors for hypogonadism in male patients with type 2 diabetes. J Diabetes Res 2016;2016:5162167.
5. Wang C, Nieschlag E, Swerdloff R, et al. International Society of Andrology (ISA). Investigation, treatment and monitoring of late-onset hypogonadism in males: ISA, ISSAM, EAU, EAA and ASA recommendations. J Androl 2009;30:1-9.
6. Santi D. ; Corona G. Primary and secondary hypogonadism. In Endocrinology of the Testis and Male Reproduction, Simoni, M., Huhtaniemi, I., Eds.Lenzi, A., Jannini, E.A., Eds.; Endocrinology; Springer; 2017. 687-747.
7. Wu FC, Tajar A, Beynon JM, et al. EMAS Group. Identification of late-onset hypogonadism in middle-aged and elderly men. N Engl J Med 2010;363:123-35.
8. Bhasin S, Brito JP, Cunningham GR, et al. Testosterone therapy in men with hypogonadism: an endocrine society clinical practice guideline. J Clin Endocrinol Metab 2018;103:1715-44.
9. Hackett G, Kirby M, Edwards D, et al. British society for sexual medicine guidelines on adult testosterone deficiency, with statements for uk practice. J Sex Med 2017;14:1504-23.
10. Salonia A, Bettocchi C, Boeri L, et al. EAU Working Group on Male Sexual and Reproductive Health. European association of urology guidelines on sexual and reproductive health-2021 update: male sexual dysfunction. Eur Urol 2021;80:333-57.
11. Wittert G, Grossmann M. Obesity, type 2 diabetes, and testosterone in ageing men. Rev Endocr Metab Disord 2022;23:1233-42.
12. Greco C, Corleto R, Ebert R, et al. Male hypogonadism and pre-diabetes interplay: association or causal interaction? a systematic review. MTOD 2021;1:11.
13. Pye SR, Huhtaniemi IT, Finn JD, et al. EMAS Study Group. Late-onset hypogonadism and mortality in aging men. J Clin Endocrinol Metab 2014;99:1357-66.
14. Andò S, Rubens R, Rottiers R. Androgen plasma levels in male diabetics. J Endocrinol Invest 1984;7:21-4.
15. Barrett-Connor E, Khaw KT, Yen SS. Endogenous sex hormone levels in older adult men with diabetes mellitus. Am J Epidemiol 1990;132:895-901.
16. Barrett-Connor E. Lower endogenous androgen levels and dyslipidemia in men with non-insulin-dependent diabetes mellitus. Ann Intern Med 1992;117:807-11.
17. Chang TC, Tung CC, Hsiao YL. Hormonal changes in elderly men with non-insulin-dependent diabetes mellitus and the hormonal relationships to abdominal adiposity. Gerontology 1994;40:260-7.
18. Defay R, Papoz L, Barny S, Bonnot-Lours S, Cacès E, Simon D; The CALedonia DIAbetes mellitus (CALDIA) study group. Hormonal status and NIDDM in the European and Melanesian populations of New Caledonia: a case-control study. Int J Obes Relat Metab Disord 1998;22:927-34.
19. Goodman-Gruen D, Barrett-Connor E. Sex differences in the association of endogenous sex hormone levels and glucose tolerance status in older men and women. Diabetes Care 2000;23:912-8.
20. Betancourt-Albrecht M, Cunningham GR. Hypogonadism and diabetes. Int J Impot Res 2003;15 Suppl 4:S14-20.
21. Tomar R, Dhindsa S, Chaudhuri A, et al. Contrasting testosterone concentrations in type 1 and type 2 diabetes. Diabetes Care 2006;29:1120-2.
22. Grossmann M, Thomas MC, Panagiotopoulos S, et al. Low testosterone levels are common and associated with insulin resistance in men with diabetes. J Clin Endocrinol Metab 2008;93:1834-40.
23. Liu RT, Chung MS, Wang PW, et al. The prevalence and predictors of androgen deficiency in Taiwanese men with type 2 diabetes. Urology 2013;82:124-9.
24. Ng Tang Fui M, Hoermann R, Cheung AS, et al. Obesity and age as dominant correlates of low testosterone in men irrespective of diabetes status. Andrology 2013;1:906-12.
25. Ho CH, Jaw FS, Wu CC, et al. The prevalence and the risk factors of testosterone deficiency in newly diagnosed and previously known type 2 diabetic men. J Sex Med 2015;12:389-97.
26. Herrero A, Marcos M, Galindo P, Miralles JM, Corrales JJ. Clinical and biochemical correlates of male hypogonadism in type 2 diabetes. Andrology 2018;6:58-63.
27. Dhindsa S, Prabhakar S, Sethi M, et al. Frequent occurrence of hypogonadotropic hypogonadism in type 2 diabetes. J Clin Endocrinol Metab 2004;89:5462-8.
28. Chandel A, Dhindsa S, Topiwala S, Chaudhuri A, Dandona P. Testosterone concentration in young patients with diabetes. Diabetes Care 2008;31:2013-7.
29. Holt SK, Lopushnyan N, Hotaling J, et al. Diabetes Control and Complications Trial/Epidemiology of Diabetes Interventions and Complications Research Group. Prevalence of low testosterone and predisposing risk factors in men with type 1 diabetes mellitus: findings from the DCCT/EDIC. J Clin Endocrinol Metab 2014;99:E1655-60.
30. Chillarón JJ, Fernández-Miró M, Albareda M, et al. TEST-DM1 Study Group. Age, insulin requirements, waist circumference, and triglycerides predict hypogonadotropic hypogonadism in patients with type 1 diabetes. J Sex Med 2015;12:76-82.
31. Cai M, Cui R, Yang P, et al. Incidence and risk factors of hypogonadism in male patients with latent autoimmune diabetes and classic type 2 diabetes. Front Endocrinol 2021;12:675525.
32. Ding EL, Song Y, Malik VS, Liu S. Sex differences of endogenous sex hormones and risk of type 2 diabetes: a systematic review and meta-analysis. JAMA 2006;295:1288-99.
33. Wang J, Yan AF, Cheskin LJ, Shi Z. Higher serum testosterone level was associated with a lower risk of prediabetes in US adults: findings from nationally representative data. Nutrients 2022;15:9.
34. Tripathy D, Dhindsa S, Garg R, et al. Hypogonadotropic hypogonadism in erectile dysfunction associated with type 2 diabetes mellitus: a common defect? Metab Syndr Relat Disord 2003;1:75-80.
35. Ali ST, Shaikh RN, Ashfaqsiddiqi N, Siddiqi PQ. Serum and urinary levels of pituitary--gonadal hormones in insulin-dependent and non-insulin-dependent diabetic males with and without neuropathy. Arch Androl 1993;30:117-23.
36. Haffner SM, Karhapää P, Mykkänen L, Laakso M. Insulin resistance, body fat distribution, and sex hormones in men. Diabetes 1994;43:212-9.
37. Simon D, Preziosi P, Barrett-Connor E, et al. Interrelation between plasma testosterone and plasma insulin in healthy adult men: the Telecom Study. Diabetologia 1992;35:173-7.
38. Tsai EC, Matsumoto AM, Fujimoto WY, Boyko EJ. Association of bioavailable, free, and total testosterone with insulin resistance: influence of sex hormone-binding globulin and body fat. Diabetes Care 2004;27:861-8.
39. Kaur H, Werstuck GH. The effect of testosterone on cardiovascular disease and cardiovascular risk factors in men: a review of clinical and preclinical data. CJC Open 2021;3:1238-48.
40. Jones TH, Kelly DM. Randomized controlled trials - mechanistic studies of testosterone and the cardiovascular system. Asian J Androl 2018;20:120-30.
41. Münzer T, Harman SM, Sorkin JD, Blackman MR. Growth hormone and sex steroid effects on serum glucose, insulin, and lipid concentrations in healthy older women and men. J Clin Endocrinol Metab 2009;94:3833-41.
42. Sato K, Iemitsu M, Aizawa K, Ajisaka R. Testosterone and DHEA activate the glucose metabolism-related signaling pathway in skeletal muscle. Am J Physiol Endocrinol Metab 2008;294:E961-8.
43. Dhindsa S, Ghanim H, Batra M, et al. Insulin resistance and inflammation in hypogonadotropic hypogonadism and their reduction after testosterone replacement in men with type 2 diabetes. Diabetes Care 2016;39:82-91.
44. Pitteloud N, Hardin M, Dwyer AA, et al. Increasing insulin resistance is associated with a decrease in Leydig cell testosterone secretion in men. J Clin Endocrinol Metab 2005;90:2636-41.
45. Paik SG, Michelis MA, Kim YT, Shin S. Induction of insulin-dependent diabetes by streptozotocin. Inhibition by estrogens and potentiation by androgens. Diabetes 1982;31:724-9.
46. Palomar-Morales M, Morimoto S, Mendoza-Rodríguez CA, Cerbón MA. The protective effect of testosterone on streptozotocin-induced apoptosis in beta cells is sex specific. Pancreas 2010;39:193-200.
47. Tirabassi G, Gioia A, Giovannini L, et al. Testosterone and cardiovascular risk. Intern Emerg Med 2013;8 Suppl 1:S65-9.
48. Tsai EC, Boyko EJ, Leonetti DL, Fujimoto WY. Low serum testosterone level as a predictor of increased visceral fat in Japanese-American men. Int J Obes Relat Metab Disord 2000;24:485-91.
49. Gupta V, Bhasin S, Guo W, et al. Effects of dihydrotestosterone on differentiation and proliferation of human mesenchymal stem cells and preadipocytes. Mol Cell Endocrinol 2008;296:32-40.
50. Tchernof A, Després JP. Pathophysiology of human visceral obesity: an update. Physiol Rev 2013;93:359-404.
51. Dieudonne MN, Pecquery R, Boumediene A, Leneveu MC, Giudicelli Y. Androgen receptors in human preadipocytes and adipocytes: regional specificities and regulation by sex steroids. Am J Physiol 1998;274:C1645-52.
52. Pecquery R, Dieudonne MN, Cloix JF, et al. Enhancement of the expression of the alpha 2-adrenoreceptor protein and mRNA by a direct effect of androgens in white adipocytes. Biochem Biophys Res Commun 1995;206:112-8.
53. Stefan N, Schick F, Birkenfeld AL, Häring HU, White MF. The role of hepatokines in NAFLD. Cell Metab 2023;35:236-52.
54. Bourebaba N, Ngo T, Śmieszek A, Bourebaba L, Marycz K. Sex hormone binding globulin as a potential drug candidate for liver-related metabolic disorders treatment. Biomed Pharmacother 2022;153:113261.
55. Rochira V, Zirilli L, Genazzani AD, et al. Hypothalamic-pituitary-gonadal axis in two men with aromatase deficiency: evidence that circulating estrogens are required at the hypothalamic level for the integrity of gonadotropin negative feedback. Eur J Endocrinol 2006;155:513-22.
56. Fink J, Matsumoto M, Tamura Y. Potential application of testosterone replacement therapy as treatment for obesity and type 2 diabetes in men. Steroids 2018;138:161-6.
57. Stefan N. Causes, consequences, and treatment of metabolically unhealthy fat distribution. Lancet Diabetes Endocrinol 2020;8:616-27.
58. Corona G, Rastrelli G, Monami M, et al. Body weight loss reverts obesity-associated hypogonadotropic hypogonadism: a systematic review and meta-analysis. Eur J Endocrinol 2013;168:829-43.
59. Lotti F, Rastrelli G, Maseroli E, et al. Impact of metabolically healthy obesity in patients with andrological problems. J Sex Med 2019;16:821-32.
60. Cohen PG. The hypogonadal-obesity cycle: role of aromatase in modulating the testosterone-estradiol shunt--a major factor in the genesis of morbid obesity. Med Hypotheses 1999;52:49-51.
61. Finn PD, Cunningham MJ, Pau KY, et al. The stimulatory effect of leptin on the neuroendocrine reproductive axis of the monkey. Endocrinology 1998;139:4652-62.
62. Majumdar S, Mukherjee JJ, Ray S, et al. Testosterone replacement therapy in men with type 2 diabetes mellitus and functional hypogonadism -an integrated diabetes and endocrine academy (IDEA) consensus guideline. Diabetes Metab Syndr 2021;15:102191.
63. Blouin K, Veilleux A, Luu-The V, Tchernof A. Androgen metabolism in adipose tissue: recent advances. Mol Cell Endocrinol 2009;301:97-103.
64. Soisson V, Brailly-Tabard S, Helmer C, et al. A J-shaped association between plasma testosterone and risk of ischemic arterial event in elderly men: the French 3C cohort study. Maturitas 2013;75:282-8.
65. Laughlin GA, Barrett-Connor E, Bergstrom J. Low serum testosterone and mortality in older men. J Clin Endocrinol Metab 2008;93:68-75.
66. Shores MM, Matsumoto AM, Sloan KL, Kivlahan DR. Low serum testosterone and mortality in male veterans. Arch Intern Med 2006;166:1660-5.
67. Khaw KT, Dowsett M, Folkerd E, et al. Endogenous testosterone and mortality due to all causes, cardiovascular disease, and cancer in men: European prospective investigation into cancer in Norfolk (EPIC-Norfolk) prospective population study. Circulation 2007;116:2694-701.
68. Corona G, Rastrelli G, Di Pasquale G, et al. Endogenous testosterone levels and cardiovascular risk: meta-analysis of observational studies. J Sex Med 2018;15:1260-71.
69. Muraleedharan V, Marsh H, Kapoor D, Channer KS, Jones TH. Testosterone deficiency is associated with increased risk of mortality and testosterone replacement improves survival in men with type 2 diabetes. Eur J Endocrinol 2013;169:725-33.
70. Vignozzi L, Gacci M, Cellai I, et al. Fat boosts, while androgen receptor activation counteracts, BPH-associated prostate inflammation. Prostate 2013;73:789-800.
71. Vignozzi L, Rastrelli G, Corona G, et al. Benign prostatic hyperplasia: a new metabolic disease? J Endocrinol Invest 2014;37:313-22.
72. Morelli A, Sarchielli E, Comeglio P, et al. Metabolic syndrome induces inflammation and impairs gonadotropin-releasing hormone neurons in the preoptic area of the hypothalamus in rabbits. Mol Cell Endocrinol 2014;382:107-19.
73. Vignozzi L, Filippi S, Comeglio P, et al. Nonalcoholic steatohepatitis as a novel player in metabolic syndrome-induced erectile dysfunction: an experimental study in the rabbit. Mol Cell Endocrinol 2014;384:143-54.
74. Rosano GM, Vitale C, Fini M. Testosterone in men with hypogonadism and high cardiovascular risk, pros. Endocrine 2015;50:320-5.
75. Keating NL, O'Malley AJ, Smith MR. Diabetes and cardiovascular disease during androgen deprivation therapy for prostate cancer. J Clin Oncol 2006;24:4448-56.
76. D'Amico AV, Denham JW, Crook J, et al. Influence of androgen suppression therapy for prostate cancer on the frequency and timing of fatal myocardial infarctions. J Clin Oncol 2007;25:2420-5.
77. Saigal CS, Gore JL, Krupski TL, Hanley J, Schonlau M, Litwin MS. Urologic Diseases in America Project. Androgen deprivation therapy increases cardiovascular morbidity in men with prostate cancer. Cancer 2007;110:1493-500.
78. Tsai HK, D'Amico AV, Sadetsky N, Chen MH, Carroll PR. Androgen deprivation therapy for localized prostate cancer and the risk of cardiovascular mortality. J Natl Cancer Inst 2007;99:1516-24.
79. Haque R, UlcickasYood M, Xu X, et al. Cardiovascular disease risk and androgen deprivation therapy in patients with localised prostate cancer: a prospective cohort study. Br J Cancer 2017;117:1233-40.
80. Corona G G, Rastrelli G, Maseroli E, Sforza A, Maggi M. Testosterone replacement therapy and cardiovascular risk: a review. World J Mens Health 2015;33:130-42.
81. Isidori AM, Giannetta E, Gianfrilli D, et al. Effects of testosterone on sexual function in men: results of a meta-analysis. Clin Endocrinol 2005;63:381-94.
82. Isidori AM, Giannetta E, Greco EA, et al. Effects of testosterone on body composition, bone metabolism and serum lipid profile in middle-aged men: a meta-analysis. Clin Endocrinol 2005;63:280-93.
83. Snyder PJ, Peachey H, Berlin JA, et al. Effects of testosterone replacement in hypogonadal men. J Clin Endocrinol Metab 2000;85:2670-7.
84. Sih R, Morley JE, Kaiser FE, et al. Testosterone replacement in older hypogonadal men: a 12-month randomized controlled trial. J Clin Endocrinol Metab 1997;82:1661-7.
85. Jones TH, Arver S, Behre HM, et al. TIMES2 Investigators. Testosterone replacement in hypogonadal men with type 2 diabetes and/or metabolic syndrome (the TIMES2 study). Diabetes Care 2011;34:828-37.
86. Jones TH, Saad F. The effects of testosterone on risk factors for, and the mediators of, the atherosclerotic process. Atherosclerosis 2009;207:318-27.
87. Spitzer M, Huang G, Basaria S, Travison TG, Bhasin S. Risks and benefits of testosterone therapy in older men. Nat Rev Endocrinol 2013;9:414-24.
88. Basaria S, Coviello AD, Travison TG, et al. Adverse events associated with testosterone administration. N Engl J Med 2010;363:109-22.
89. Finkle WD, Greenland S, Ridgeway GK, et al. Increased risk of non-fatal myocardial infarction following testosterone therapy prescription in men. PLoS One 2014;9:e85805.
90. Vigen R, O'Donnell CI, Barón AE, et al. Association of testosterone therapy with mortality, myocardial infarction, and stroke in men with low testosterone levels. JAMA 2013;310:1829-36.
91. Corona G, Rastrelli G, Guaraldi F, et al. An update on heart disease risk associated with testosterone boosting medications. Expert Opin Drug Saf 2019;18:321-32.
92. Pugh PJ, Jones TH, Channer KS. Acute haemodynamic effects of testosterone in men with chronic heart failure. Eur Heart J 2003;24:909-15.
93. Malkin CJ, Pugh PJ, West JN, van Beek EJ, Jones TH, Channer KS. Testosterone therapy in men with moderate severity heart failure: a double-blind randomized placebo controlled trial. Eur Heart J 2006;27:57-64.
94. Caminiti G, Volterrani M, Iellamo F, et al. Effect of long-acting testosterone treatment on functional exercise capacity, skeletal muscle performance, insulin resistance, and baroreflex sensitivity in elderly patients with chronic heart failure a double-blind, placebo-controlled, randomized study. J Am Coll Cardiol 2009;54:919-27.
95. Traish AM, Haider A, Haider KS, Doros G, Saad F. Long-term testosterone therapy improves cardiometabolic function and reduces risk of cardiovascular disease in men with hypogonadism: a real-life observational registry study setting comparing treated and untreated (Control) groups. J Cardiovasc Pharmacol Ther 2017;22:414-33.
96. Bhasin S, Lincoff AM, Basaria S, et al. TRAVERSE Study Investigators. Effects of long-term testosterone treatment on cardiovascular outcomes in men with hypogonadism: Rationale and design of the TRAVERSE study. Am Heart J 2022;245:41-50.
97. Dandona P, Dhindsa S, Ghanim H, Saad F. Mechanisms underlying the metabolic actions of testosterone in humans: a narrative review. Diabetes Obes Metab 2021;23:18-28.
98. Haider KS, Haider A, Saad F, et al. Remission of type 2 diabetes following long-term treatment with injectable testosterone undecanoate in patients with hypogonadism and type 2 diabetes: 11-year data from a real-world registry study. Diabetes Obes Metab 2020;22:2055-68.
99. Wittert G, Bracken K, Robledo KP, et al. Testosterone treatment to prevent or revert type 2 diabetes in men enrolled in a lifestyle programme (T4DM): a randomised, double-blind, placebo-controlled, 2-year, phase 3b trial. Lancet Diabetes Endocrinol 2021;9:32-45.
100. Caliber M, Saad F. Testosterone therapy for prevention and reversal of type 2 diabetes in men with low testosterone. Curr Opin Pharmacol 2021;58:83-9.
101. Kumar S, Khatri M, Memon RA, et al. Effects of testosterone therapy in adult males with hypogonadism and T2DM: a meta-analysis and systematic review. Diabetes Metab Syndr 2022;16:102588.
102. Janjgava S, Zerekidze T, Uchava L, Giorgadze E, Asatiani K. Influence of testosterone replacement therapy on metabolic disorders in male patients with type 2 diabetes mellitus and androgen deficiency. Eur J Med Res 2014;19:56.
103. Jeong SM, Ham BK, Park MG, et al. Effect of testosterone replacement treatment in testosterone deficiency syndrome patients with metabolic syndrome. Korean J Urol 2011;52:566-71.
104. Ghanim H, Dhindsa S, Batra M, et al. Testosterone increases the expression and phosphorylation of amp kinase α in men with hypogonadism and type 2 diabetes. J Clin Endocrinol Metab 2020;105:1169-75.
105. Mårin P, Holmäng S, Gustafsson C, et al. Androgen treatment of abdominally obese men. Obes Res 1993;1:245-51.
106. Hackett G. Metabolic effects of testosterone therapy in men with type 2 diabetes and metabolic syndrome. Sex Med Rev 2019;7:476-90.
107. Hackett G, Cole N, Bhartia M, Kennedy D, Raju J, Wilkinson P. BLAST Study Group. Testosterone replacement therapy improves metabolic parameters in hypogonadal men with type 2 diabetes but not in men with coexisting depression: the BLAST study. J Sex Med 2014;11:840-56.
108. Krysiak R, Gilowski W, Okopień B. The effect of testosterone on cardiovascular risk factors in men with type 2 diabetes and late-onset hypogonadism treated with metformin or glimepiride. Pharmacol Rep 2016;68:75-9.
109. Groti K, Žuran I, Antonič B, Foršnarič L, Pfeifer M. The impact of testosterone replacement therapy on glycemic control, vascular function, and components of the metabolic syndrome in obese hypogonadal men with type 2 diabetes. Aging Male 2018;21:158-69.
110. Khripun I, Vorobyev S, Belousov I, Kogan M, Zitzmann M. Influence of testosterone substitution on glycemic control and endothelial markers in men with newly diagnosed functional hypogonadism and type 2 diabetes mellitus: a randomized controlled trial. Aging Male 2019;22:241-9.
111. Farias JM, Tinetti M, Khoury M, Umpierrez GE. Low testosterone concentration and atherosclerotic disease markers in male patients with type 2 diabetes. J Clin Endocrinol Metab 2014;99:4698-703.
112. Daka B, Langer RD, Larsson CA, et al. Low concentrations of serum testosterone predict acute myocardial infarction in men with type 2 diabetes mellitus. BMC Endocr Disord 2015;15:35.
115. Rosenstock J, Chuck L, González-Ortiz M, et al. Initial combination therapy with canagliflozin plus metformin versus each component as monotherapy for drug-naïve type 2 diabetes. Diabetes Care 2016;39:353-62.
116. Søfteland E, Meier JJ, Vangen B, et al. Empagliflozin as add-on therapy in patients with type 2 diabetes inadequately controlled with linagliptin and metformin: a 24-week randomized, double-blind, parallel-group trial. Diabetes Care 2017;40:201-9.
117. Faure M, Guibert E, Alves S, et al. The insulin sensitiser metformin regulates chicken Sertoli and germ cell populations. Reproduction 2016;151:527-38.
118. Tartarin P, Moison D, Guibert E, et al. Metformin exposure affects human and mouse fetal testicular cells. Hum Reprod 2012;27:3304-14.
119. Hu Y, Ding B, Shen Y, et al. Rapid Changes in Serum Testosterone in men with newly diagnosed type 2 diabetes with intensive insulin and metformin. Diabetes Care 2021;44:1059-61.
120. Cai T, Hu Y, Ding B, et al. Effect of metformin on testosterone levels in male patients with type 2 diabetes mellitus treated with insulin. Front Endocrinol 2021;12:813067.
121. Martin Martins J, de Pina Jorge M, Martins Maia C, et al. Primary and secondary hypogonadism in male persons with diabetes mellitus. Int J Endocrinol 2021;2021:8799537.
122. Wensink MJ, Lu Y, Tian L, et al. Preconception antidiabetic drugs in men and birth defects in offspring : a nationwide cohort study. Ann Intern Med 2022;175:665-73.
123. Caruso I, Cignarelli A, Laviola L, Giorgino F. GLP-1 receptor agonists for cardiovascular protection: a matter of time. Diabetes Care 2022;45:e30-1.
124. Wadden TA, Hollander P, Klein S, et al. NN8022-1923 Investigators. Weight maintenance and additional weight loss with liraglutide after low-calorie-diet-induced weight loss: the SCALE Maintenance randomized study. Int J Obes 2013;37:1443-51.
125. Davies MJ, Bergenstal R, Bode B, et al. NN8022-1922 Study Group. Efficacy of liraglutide for weight loss among patients with type 2 diabetes: the SCALE diabetes randomized clinical trial. JAMA 2015;314:687-99.
126. Brown E, Heerspink HJL, Cuthbertson DJ, Wilding JPH. SGLT2 inhibitors and GLP-1 receptor agonists: established and emerging indications. Lancet 2021;398:262-76.
127. Caruso I, Cignarelli A, Giorgino F. Heterogeneity and similarities in GLP-1 receptor agonist cardiovascular outcomes trials. Trends Endocrinol Metab 2019;30:578-89.
128. Caruso I, Giorgino F. SGLT-2 inhibitors as cardio-renal protective agents. Metabolism 2022;127:154937.
129. Caruso I, Cignarelli A, Laviola L, Giorgino F. Sodium-glucose co-transporter-2 inhibitors and protection from cardiovascular death: Is it all about heart failure? Diabetes Obes Metab 2021;23:2194-6.
130. Zou CY, Liu XK, Sang YQ, Wang B, Liang J. Effects of SGLT2 inhibitors on cardiovascular outcomes and mortality in type 2 diabetes: a meta-analysis. Medicine 2019;98:e18245.
131. Johansen ME, Argyropoulos C. The cardiovascular outcomes, heart failure and kidney disease trials tell that the time to use Sodium Glucose Cotransporter 2 inhibitors is now. Clin Cardiol 2020;43:1376-87.
132. Cignarelli A, Genchi VA, D'Oria R, et al. Role of glucose-lowering medications in erectile dysfunction. J Clin Med 2021;10:2501.
133. Sansone A, Rastrelli G, Cignarelli A, et al. Effect of treatment with testosterone on endothelial function in hypogonadal men: a systematic review and meta-analysis. Int J Impot Res 2020;32:379-86.
134. Efesoy O, Çayan S, Akbay E. The effect of testosterone replacement therapy on penile hemodynamics in hypogonadal men with erectile dysfunction, having veno-occlusive dysfunction. Am J Mens Health 2018;12:634-8.
135. Morgentaler A, Miner MM, Caliber M, Guay AT, Khera M, Traish AM. Testosterone therapy and cardiovascular risk: advances and controversies. Mayo Clin Proc 2015;90:224-51.
136. Morgentaler A, Zitzmann M, Traish AM, et al. Fundamental concepts regarding testosterone deficiency and treatment: international expert consensus resolutions. Mayo Clin Proc 2016;91:881-96.
137. Wallis CJ, Lo K, Lee Y, et al. Survival and cardiovascular events in men treated with testosterone replacement therapy: an intention-to-treat observational cohort study. Lancet Diabetes Endocrinol 2016;4:498-506.
138. MacLusky NJ, Cook S, Scrocchi L, et al. Neuroendocrine function and response to stress in mice with complete disruption of glucagon-like peptide-1 receptor signaling. Endocrinology 2000;141:752-62.
139. Caltabiano R, Condorelli D, Panza S, et al. Glucagon-like peptide-1 receptor is expressed in human and rodent testis. Andrology 2020;8:1935-45.
140. Beak SA, Heath MM, Small CJ, et al. Glucagon-like peptide-1 stimulates luteinizing hormone-releasing hormone secretion in a rodent hypothalamic neuronal cell line. J Clin Invest 1998;101:1334-41.
141. Oride A, Kanasaki H, Mijiddorj T, et al. GLP-1 increases Kiss-1 mRNA expression in kisspeptin-expressing neuronal cells. Biol Reprod 2017;97:240-8.
142. Abdullah DM, Alsemeh AE, Khamis T. Semaglutide early intervention attenuated testicular dysfunction by targeting the GLP-1-PPAR-α-Kisspeptin-Steroidogenesis signaling pathway in a testicular ischemia-reperfusion rat model. Peptides 2022;149:170711.
143. Zhang E, Xu F, Liang H, et al. GLP-1 receptor agonist exenatide attenuates the detrimental effects of obesity on inflammatory profile in testis and sperm quality in mice. Am J Reprod Immunol 2015;74:457-66.
144. Martins AD, Monteiro MP, Silva BM, et al. Metabolic dynamics of human Sertoli cells are differentially modulated by physiological and pharmacological concentrations of GLP-1. Toxicol Appl Pharmacol 2019;362:1-8.
145. Rago V, De Rose D, Santoro M, et al. Human sperm express the receptor for glucagon-like peptide-1 (GLP-1), which affects sperm function and metabolism. Endocrinology 2020;161:bqaa031.
146. Marso SP, Daniels GH, Brown-Frandsen K, et al. LEADER Steering Committee. Liraglutide and cardiovascular outcomes in type 2 diabetes. N Engl J Med 2016;375:311-22.
147. Gerstein HC, Colhoun HM, Dagenais GR, et al. REWIND Investigators. Dulaglutide and cardiovascular outcomes in type 2 diabetes (REWIND): a double-blind, randomised placebo-controlled trial. Lancet 2019;394:121-30.
148. Marso SP, Bain SC, Consoli A, et al. SUSTAIN-6 Investigators. Semaglutide and cardiovascular outcomes in patients with type 2 diabetes. N Engl J Med 2016;375:1834-44.
149. Holman RR, Bethel MA, George J, et al. Rationale and design of the EXenatide study of cardiovascular event lowering (EXSCEL) trial. Am Heart J 2016;174:103-10.
150. Holman RR, Bethel MA, Mentz RJ, et al. EXSCEL Study Group. Effects of once-weekly exenatide on cardiovascular outcomes in type 2 diabetes. N Engl J Med 2017;377:1228-39.
151. Bethel MA, Patel RA, Merrill P, et al. EXSCEL Study Group. Cardiovascular outcomes with glucagon-like peptide-1 receptor agonists in patients with type 2 diabetes: a meta-analysis. Lancet Diabetes Endocrinol 2018;6:105-13.
152. Sudo M, Li Y, Hiro T, et al. Inhibition of plaque progression and promotion of plaque stability by glucagon-like peptide-1 receptor agonist: Serial
153. Yuan P, Ma D, Gao X, et al. Liraglutide ameliorates erectile dysfunction via regulating oxidative stress, the RhoA/ROCK pathway and autophagy in diabetes mellitus. Front Pharmacol 2020;11:1257.
154. Giagulli VA, Carbone MD, Ramunni MI, et al. Adding liraglutide to lifestyle changes, metformin and testosterone therapy boosts erectile function in diabetic obese men with overt hypogonadism. Andrology 2015;3:1094-103.
155. Bajaj HS, Gerstein HC, Rao-Melacini P, et al. Erectile function in men with type 2 diabetes treated with dulaglutide: an exploratory analysis of the REWIND placebo-controlled randomised trial. Lancet Diabetes Endocrinol 2021;9:484-90.
156. Shao N, Yu XY, Yu YM, et al. Short-term combined treatment with exenatide and metformin is superior to glimepiride combined metformin in improvement of serum testosterone levels in type 2 diabetic patients with obesity. Andrologia 2018;50:e13039.
157. Jensterle M, Podbregar A, Goricar K, Gregoric N, Janez A. Effects of liraglutide on obesity-associated functional hypogonadism in men. Endocr Connect 2019;8:195-202.
158. Giagulli VA, Castellana M, Carbone MD, et al. Weight loss more than glycemic control may improve testosterone in obese type 2 diabetes mellitus men with hypogonadism. Andrology 2020;8:654-62.
159. Graybill S, Hatfield J, Kravchenko M, et al. Neutral effect of exenatide on serum testosterone in men with type 2 diabetes mellitus: a prospective cohort. Andrology 2021;9:792-800.
160. Jeibmann A, Zahedi S, Simoni M, Nieschlag E, Byrne MM. Glucagon-like peptide-1 reduces the pulsatile component of testosterone secretion in healthy males. Eur J Clin Invest 2005;35:565-72.
161. Lee DM, Battson ML, Jarrell DK, et al. SGLT2 inhibition via dapagliflozin improves generalized vascular dysfunction and alters the gut microbiota in type 2 diabetic mice. Cardiovasc Diabetol 2018;17:62.
162. Park SH, Farooq MA, Gaertner S, et al. Empagliflozin improved systolic blood pressure, endothelial dysfunction and heart remodeling in the metabolic syndrome ZSF1 rat. Cardiovasc Diabetol 2020;19:19.
163. Solini A, Giannini L, Seghieri M, et al. Dapagliflozin acutely improves endothelial dysfunction, reduces aortic stiffness and renal resistive index in type 2 diabetic patients: a pilot study. Cardiovasc Diabetol 2017;16:138.
164. Assaly R, Gorny D, Compagnie S, et al. The favorable effect of empagliflozin on erectile function in an experimental model of type 2 diabetes. J Sex Med 2018;15:1224-34.
165. Luo ZC, Jin ZR, Jiang YF, et al. The protective effects and underlying mechanisms of dapagliflozin on diabetes-induced testicular dysfunction. Asian J Androl 2023;25:331-8.
166. Araujo AB, Dixon JM, Suarez EA, Murad MH, Guey LT, Wittert GA. Endogenous testosterone and mortality in men: a systematic review and meta-analysis. J Clin Endocrinol Metab 2011;96:3007-19.
167. Gagliano-Jucá T, Basaria S. Testosterone replacement therapy and cardiovascular risk. Nat Rev Cardiol 2019;16:555-74.
168. Malipatil NS, Yadegarfar G, Lunt M, et al. Male hypogonadism: 14-year prospective outcome in 550 men with type 2 diabetes. Endocrinol Diabetes Metab 2019;2:e00064.
169. Yao QM, Wang B, An XF, Zhang JA, Ding L. Testosterone level and risk of type 2 diabetes in men: a systematic review and meta-analysis. Endocr Connect 2018;7:220-31.
170. Kapoor D, Goodwin E, Channer KS, Jones TH. Testosterone replacement therapy improves insulin resistance, glycaemic control, visceral adiposity and hypercholesterolaemia in hypogonadal men with type 2 diabetes. Eur J Endocrinol 2006;154:899-906.
171. Gianatti EJ, Dupuis P, Hoermann R, et al. Effect of testosterone treatment on glucose metabolism in men with type 2 diabetes: a randomized controlled trial. Diabetes Care 2014;37:2098-107.
Cite This Article
Export citation file: BibTeX | RIS
OAE Style
Genchi VA, Zanni E, Colzani M, Lauriola C, Cignarelli A, Santi D, Greco C. New antidiabetic drugs' role in the management of testosterone deficiency and of the cardiovascular disease in hypogonadal diabetic men. Metab Target Organ Damage 2023;3:8. http://dx.doi.org/10.20517/mtod.2023.06
AMA Style
Genchi VA, Zanni E, Colzani M, Lauriola C, Cignarelli A, Santi D, Greco C. New antidiabetic drugs' role in the management of testosterone deficiency and of the cardiovascular disease in hypogonadal diabetic men. Metabolism and Target Organ Damage. 2023; 3(2): 8. http://dx.doi.org/10.20517/mtod.2023.06
Chicago/Turabian Style
Genchi, Valentina Annamaria, Eleonora Zanni, Massimiliano Colzani, Celeste Lauriola, Angelo Cignarelli, Daniele Santi, Carla Greco. 2023. "New antidiabetic drugs' role in the management of testosterone deficiency and of the cardiovascular disease in hypogonadal diabetic men" Metabolism and Target Organ Damage. 3, no.2: 8. http://dx.doi.org/10.20517/mtod.2023.06
ACS Style
Genchi, VA.; Zanni E.; Colzani M.; Lauriola C.; Cignarelli A.; Santi D.; Greco C. New antidiabetic drugs' role in the management of testosterone deficiency and of the cardiovascular disease in hypogonadal diabetic men. Metab Target Organ Damage. 2023, 3, 8. http://dx.doi.org/10.20517/mtod.2023.06
About This Article
Special Issue
Copyright
Data & Comments
Data
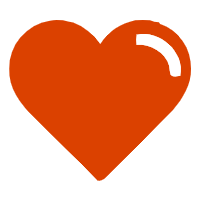

Comments
Comments must be written in English. Spam, offensive content, impersonation, and private information will not be permitted. If any comment is reported and identified as inappropriate content by OAE staff, the comment will be removed without notice. If you have any queries or need any help, please contact us at support@oaepublish.com.